The kind of problem a building is
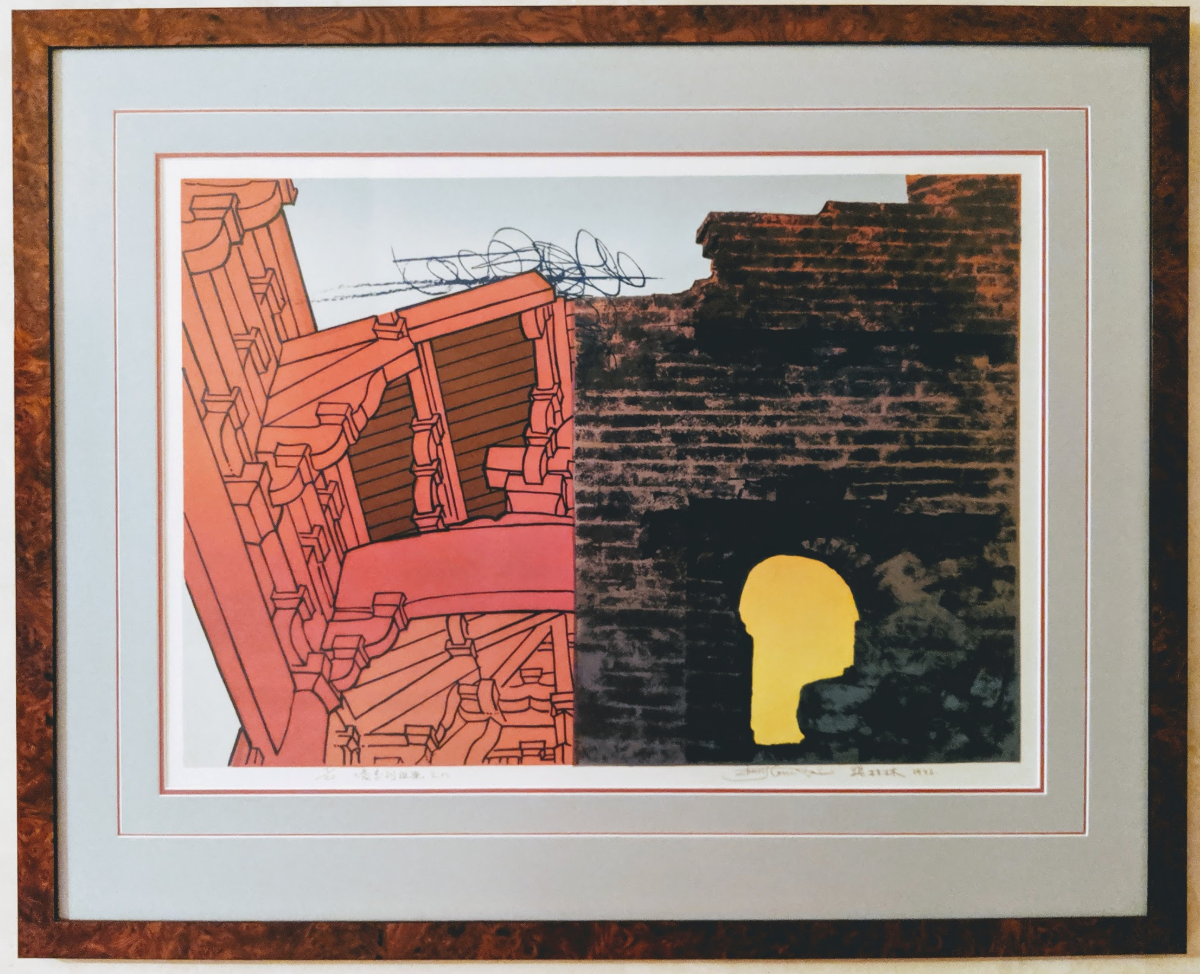
Concluding her famous book, Jane Jacobs writes: “Cities happen to be problems in organized complexity.” From sidewalks of access to sidewalks of place, cities deal “simultaneously with a sizable number of factors which are interrelated into an organic whole [1].”
My question today: If a city is a problem of organized complexity, then what kind of problem is a building?
Part 1. Core & Shell
The life of buildings
Buildings are where we spend most of our time: we spend at least 80% of our time indoors. In addition to our time, they consume much of our energy. Buildings use 30% of global end use energy for things like space heating, cooling, and lighting– and that excludes the energy to make construction materials.
Think about your own experience with buildings. It may be your home, the civic institution you frequent, the structure you seek shade in, the shape you view along the skyline, or the place you contribute your work in. We interact with buildings of all types. We intuitively know their forms and functions.
The life of buildings is not a concept reserved for building engineers and architects. “The processes that occur in cities are not arcane, capable of being understood only by experts. They can be understood by almost anybody,” Jacobs writes. So too, for buildings. At 80% of our time, shouldn’t we also be able to understand their processes?
Seeds of organized complexity
When she wrote about organized complexity, Jacobs was citing Warren Weaver– a mathematician, science administrator… and Alice in Wonderland aficionado .
Weaver first wrote about organized complexity in a 1948 American Scientist article titled “ Science and Complexity“. His key point: that a focus on “organized” complexity, the grey area between simple problems of limited variables and disorganized problems (those that can be solved with statistics) can generate the most opportunity. This insight came from his years working in science development. A teacher and communicator, Weaver was one of the patrons of U.S. science during and in the years following WWII. As Director of the Natural Sciences division at the Rockefeller Foundation, his directive was to “support the well-being of mankind throughout the world [2].” He supported and funded scientists who went on to establish new disciplines like information theory and molecular biology.
The main focus of Weaver’s work was to move biology towards “more modern experimental studies […] as contrasted with the older collecting and descriptive studies.” The bulk of his funding was in the new world of experimental biology, a focus on the life sciences rather than the physical sciences.
That being said, he contributed to a variety of projects across mixed teams and disciplines. Here are just a few of the projects Warren Weaver supported:
- The Differential Analyzer, Vannevar Bush (1935)– though the project was a short-lived analog computer, Weaver still thought it had import for its educational value… and most certainly it did, given that both Bush and Claude Shannon spent their early careers on it [3]
- Study of predictive feedback loops, Norbert Wiener (concluded 1942)– this research became The Extrapolation, Interpolation, and Smoothing of Stationary Time Series and ultimately influenced Wiener’s cybernetics work
- The Dartmouth Conference, John McCarthy (1956)– while he wasn’t part of the final decision (he felt too personally involved given Claude Shannon’s attendance to the conference), Weaver played an earlier role in the vetting process of the first Artificial Intelligence gathering
- Projects in language translation, operations research, game theory, etc [4]
In other words: this work had reach.
Perhaps what I find most remarkable about Warren Weaver was his ability to take complicated ideas from scientists and translate them into more common understanding, making them accessible to larger audiences. The best known example is The Mathematical Theory of Communication(1949), a reprinting of Claude Shannon’s technical paper from the Bell System Technical Journal as a standalone book (with article about-face [5]) and supplemental lay-person introduction by Weaver. This book decidedly gave the new concept of information theory a wider reach.
Of systems and cities
Warren Weaver’s concept of organized complexity went on to influence several voices in the systems and cities worlds; Jacobs was not alone. Herbert Simon, a systems thinker (among many other titles), cites Weaver and his American Scientist piece as a key influence in his phenomenal essay “ The Architecture of Complexity.” In this piece Simon makes the case that systems are hierarchical, an ordering of stable subsystems. His version of complexity was clearly that of the organized form.
Jane Jacobs herself was influenced by a reprint of Warren Weaver’s “Science and Complexity” article, an expansion made available in the 1958 Annual Report of the Rockefeller Foundation. While Weaver was writing about the life sciences, Jacobs believed that his vision of organized complexity could just as suitably be applied to cities. “The recent progress of the life sciences tells us something tremendously important about other problems of organized complexity. It tells us that problems of this kind can be analyzed– that it is only sensible to regard them as capable of being understood” instead of thinking of them as some “irrational” force. She makes the point that cities, too, can be understood.
Part 2. Fit Out
Close your eyes. Imagine that you’re in a building. Reflect on what this building is like. What do you sense? What do you feel? What do you think?
I’ll put a wager out there; you’re likely not thinking about the building itself. Rather, you’re thinking about your experience within it. Shaped and formed in minute ways that we still probably cannot understand [6]. You’re thinking of the sense and feeling of the place, either a specific moment or an accumulation of moments, rather than the master planned vision [7].
Sometimes these feelings of place are instrumented by the people around us. And sometimes, these experiences of place are instrumented, often in concert, by the systems involved. Buildings are the container for both.
Building blocks of feedback loops
Whenever a systems writer begins to illustrate the concept of systems thinking, he or she starts with a simple building subsystem: the thermostat, or more specifically, a room thermostat [8].
A thermostat is the classic example of a negative (balancing) feedback loop. A goal state is identified, a desired temperature setting in this case. Sensors read the current state, the temperature of the room, and the system error-corrects in the direction of the goal. The room temperature changes and the process continues.
Norbert Wiener highlights thermostats as negative feedback loops to stabilize temperature in his 1948 book on cybernetics.
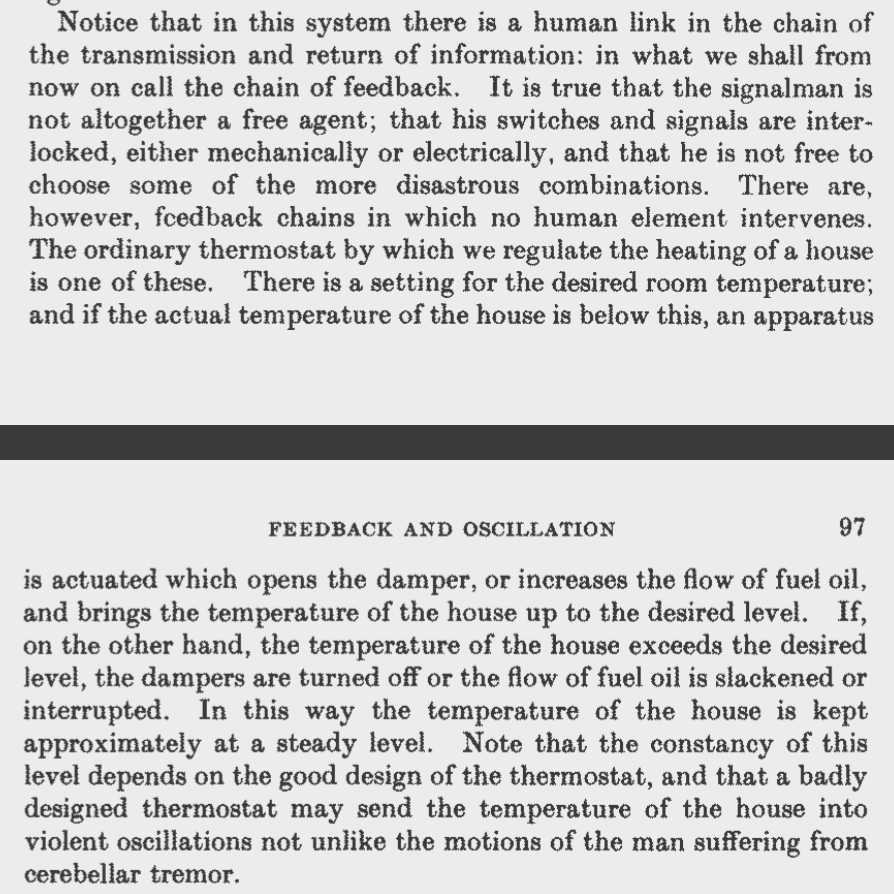
Cybernetics: or Control and Communication in the Animal and the Machine
Donella Meadows uses a thermostat example to describe competing balancing feedback loops, like in the example shown below. One feedback loop is related to the heat input from a furnace, and the other is related to heat output from leaks out of the room.
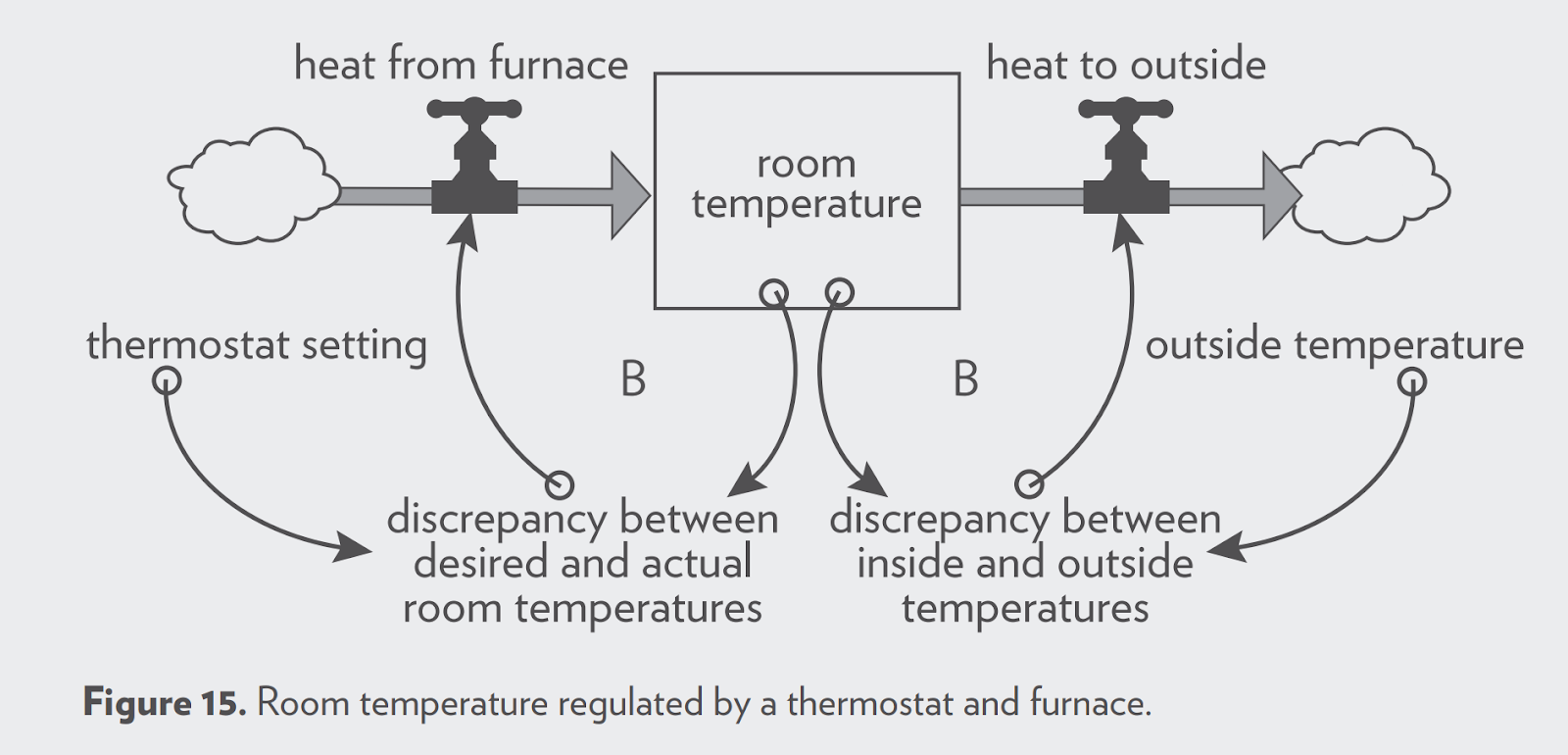
Ross Ashby writes about thermostats in his 1958 piece on Requisite Variety, describing the error correction of thermostat regulators as “noise” and the desired setpoint, or goal, as “zero entropy.”
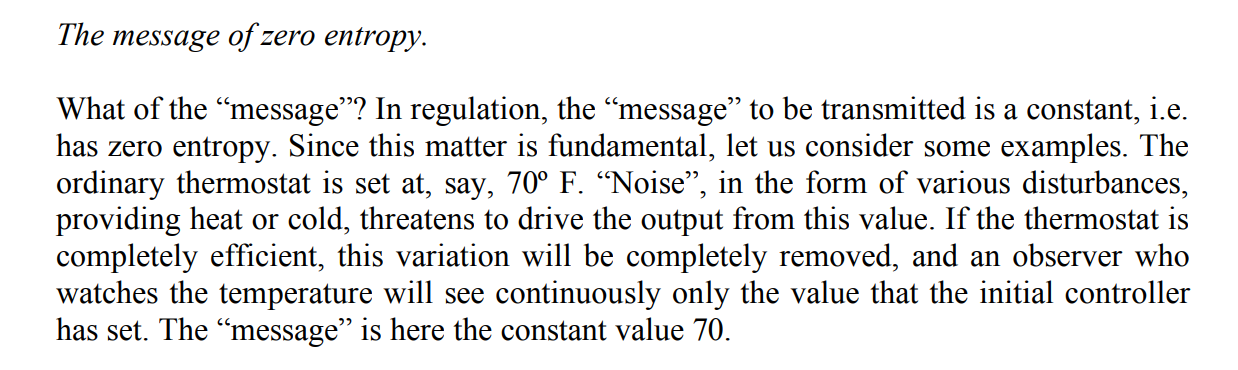
Requisite variety and its implications for the control of complex systems
And Herb Simon takes this a step further in “The Architecture of Complexity” to go beyond temperature regulation to discuss short vs. long run equilibria in nearly decomposable systems.
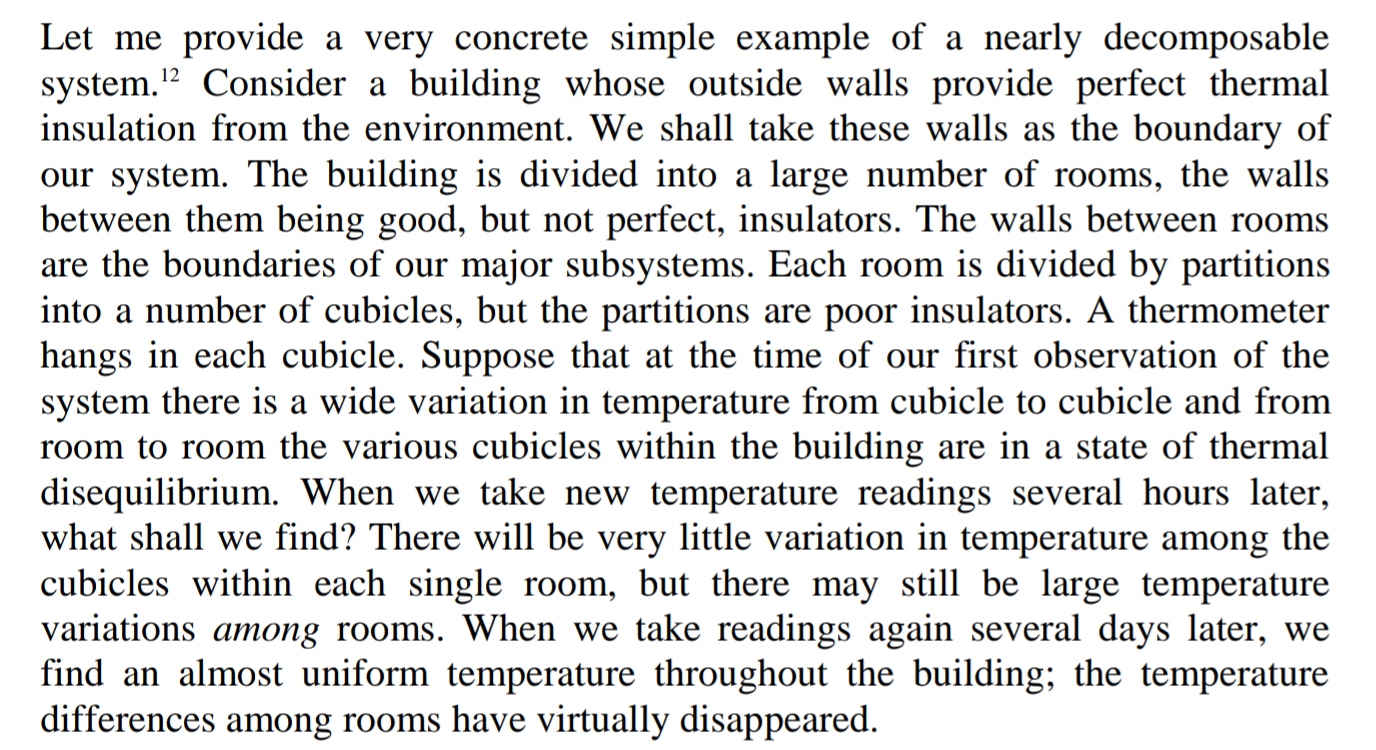
The Architecture of Complexity
Most of us live among thermostats every day. The simplest form of regulation, an expression of communication and control, they have immense impact to our indoor lives.
Patented origins
Today we might think of thermostats as just some IoT-riddled wall-mounted box– like a Nest– but room thermostats got their start in the late 1880’s. Patents by three separate founders emerged into the three companies that today still hold market dominance of the building control industry, almost 150 years later.
One of the first patents came from an invention in the basement of a school building outside of Milwaukee, Wisconsin. (Remember: Warren Weaver was from Wisconsin, too!). There, another Warren, Warren S. Johnson, invented one of the first thermostats– a device that transmitted readings from decentralized indicators throughout a building. These “indicators” rang a bell to alert the basement-bound furnace attendant to open or close the furnace dampers.
The 1883 version was still fairly manual; in 1895 Johnson released a more automated version for commercial application. This version used pneumatic controls to operate a pilot regulator, thereby eliminating the need for an attendant to control the system. Today his company, Johnson Controls, still has strong market share in the building controls industry.
At the same time Alfred Butz, a Swiss immigrant, patented the “damper flapper” in 1885, a device that would take a reading from one or multiple thermostats and then fan the flames of the furnace to increase the heat. His company is now known as Honeywell today, a key competitor to Johnson Controls.
the infamous damper flapper
In 1890 another thermostat patent was granted to an inventor, this time to William P Powers– also of Wisconsin. He went on to found the Powers Regulator Company, which–by way of an acquisition by Swiss firm Landis and Gyr–ultimately became part of Siemens Building Technologies. Today, Siemens is another major player in the building automation market.
These early thermostats, indicators of communication and control, became the key enablers to living in the conditioned indoors: a clear input in our capacity for today’s urbanization. Room thermostats expanded to further developments in building controls and automation and is a field that still powers the buildings around you, behind the scenes, every day.
What goes on in a building
As robust and commonplace building controls might seem– operating systems are an expected component in today’s devices– you may be surprised by how fragmented the industry is.
In the conventional framework, once a building investor decides to build a building they’ll first work with an architect to design their desired expanse. The architect then works with a variety of consultants– structural, mechanical, lighting, landscape– to develop the rest of the project. From there, a General Contractor (GC) is selected to coordinate the project’s construction. It’s not a surprise that most often the lowest bidder wins. The GC will then subcontract to a variety of vendors for specified systems. Fragmentation is the name of the game. Building controls for heating, ventilation, and air-conditioning (HVAC) are designed and delivered by a *different* vendor than the actual HVAC equipment. Lighting controls are designed and delivered– often, not always– by a *different* vendor than the lighting fixtures. Value engineering runs rampant and smart designs are thrown out at the last minute. Most system vendors maintain relationships with the General Contractor; after project handoff to the Building Owner, connection to post-occupancy tenants is lost. Along with this dizzy of misaligned incentives, timescales and ownership are misaligned, too.
Can you imagine if a building was an aircraft?
Building automation is a pace layer of control, ceded out to different players across the building landscape. Hidden behind the scenes, it sits beyond notice of most occupants, its only touchpoint the thermostat on the wall. But the reality is intriguing. As described above, a building owner is only responsible for the primary control systems of a building– fire control systems, elevator systems, egress lighting, often a base layer of HVAC. From there, tenants are in charge of their own fit-out. This covers interior design but can also include lighting system and HVAC upgrades. As more smart systems get integrated into our built environments, whether as additional sensors or actuators with their own control commands, these layers grow. Each new system is contracted by a different vendor. Ownership varies, suite by suite. Systems vary suite by suite. And the timescales of each of these systems and owners vary, suite by suite.
Part 3. Maintenance
Buildings after they’re built
The truth is, a building really becomes a building for the 80 years AFTER it is built. That’s when the people come– buildings are the consumer products with the longest life cycle after all. That’s also when the costs come; it’s a common industry adage that about 80% of a buildings lifecycle costs go to operations and maintenance. And it’s in this phase, post-occupancy, that the true value is generated. Businesses are built, widgets are made, lives are made healthy, and coordination among people cycles through.
When systems are in the background, they go unnoticed. When not used, deterioration sets in. As Stewart Brand writes in How Buildings Learn, “Once attention is deferred, deferring of maintenance comes naturally.” The key is to bring uses to the forefront. To connect to the actual people a system serves.
I spend a lot of my days thinking about how I can adapt the building I work in to better suit my (and my colleagues’) needs. We start by bringing the background systems to the forefront. We try to understand the systems installed– the vendors, the settings, the data points captured. We try to control systems where we can. (Deploying code on Github to run building system command sequences is pretty cool!) We try to link memory palaces and context gardens to physical spaces. I wonder about things like: How can the built world help us collaborate better? How can I better understand my movement through a space and what it says about my best ways of work? How can I virtually tour my remote colleagues’ workspaces, to perhaps understand them better?
This all seems fine on paper… until you try it.
Earlier this year, I tried to enact many of the musings I list above. I had the connections with systems vendors. I had the technology to control the systems. I knew the memory palaces I wanted to build, and the context gardens I wanted to grow. The problem was: management overhead. From our landlord to our local contractor, the refrain became: “there’s too much overhead cost to figure that out, I don’t want to do it.” We wanted to pay, but incentives were not aligned. The industry is not incentivized to make adaptations easy. As Brand hints, “Almost no buildings adapt well. They’re designed not to adapt; also budgeted and financed not to, constructed not to, administered not to, maintained not to, regulated and taxed not to, even remodeled not to.”
What worries me is that this overhead is what prevents new, impactful technologies from entering the scene. Maintenance means keeping an eye on all the pace layers, from the short term to the long term. And not letting the long term layers deteriorate. But this is easier said than done. “The management overhead is too high” goes the phrase. It reverberates.
Cybernetics of maintenance
Cybernetics is inherently about maintenance. It is about ongoing feedback loops: taking in current information and adjusting or upgrading status to a desired state.
Cybernetics is the study of control and communication, concepts connected to one another through feedback. Cybernetics had its heyday in the 1950’s and ’60s, peppered with three different flavors.
The first was Norbert Wiener’s version of cybernetics: the application of control theory to biology. The founder of the concept [9], Wiener thought that cybernetics required a robust mathematical framework and didn’t appreciate applying cybernetics to the social sciences. Enter flavor two: cybernetics actually applied to the social sciences, much to Wiener’s chagrin. Championed by the anthropologist Margaret Mead among others, their version called for the use of “cybernetic feedback circuits to model human decision making and social interactions in management science, psychology, sociology, political science, and anthropology [10].”
The third version was even less mathematical but no less resonant. As Ronald Kline writes in The Cybernetics Moment, “Second order cybernetics was a far cry from the scientific realism held by all early cyberneticians. It resonates instead with the associations between cybernetics and counterculture.” In second order cybernetics, the emphasis was on observing behavior: the observer can not discount herself from the system in which she participates.
Christopher Alexander, architect of patterns, echoed second order cybernetics when he wrote: “This is exactly what happens when a systems analyst looks at a building: he/she manages to describe the circulation, the acoustics, the heating and the load-bearing structure as systems– and fails to identify the most interesting human and social systems, because he/she cant describe them in explicit terms.”
For all of the focus on the fragmentation of building systems, what is missing is how these systems interact with the primary end users: the occupants of a building. The most interesting human and social systems. As an observer to the whole system, one cannot extract oneself from the system; we are a part of it. This is second order cybernetics. This is where organized complexity lies.
What’s the point?
To quote Herbert Simon, “Our speculations have carried us over a rather alarming array of topics, but that is the price we must pay if we wish to seek properties common to many sorts of complex systems.”
“The theorists of conventional modern city planning have consistently mistaken cities as problems of simplicity and of disorganized complexity, and have tried to analyze and treat them thus,” Jacobs confides. Similarly, the buildings industry has mistaken buildings as problems of simplicity and of disorganized complexity.
A new way is possible to think about the design, build, and operation of our buildings. But it also has to do with organization and incentives rather than just updated building codes, new tools for design, or more efficient materials for construction [11]. What are some of these opportunities? Reducing overhead costs due to fragmentation of information. Realigning the costs and benefits for those who design or build vs. exist in a building. Understanding the impact that built environments have on our senses– from how we feel, to how we experience things, to how we coordinate– to spur more attention at the “end user” level.
In the same way that JJ wrote about organized complexity in cities to vocalize a new version of urbanism, I’m advocating for a new way of thinking about buildings. The dire situation is this: the infrastructure surrounding buildings is constrained. Over 30% of building energy can be wasted by just not paying attention to maintenance. New technologies exist with costs going down, but how this tech gets into buildings remains an open question. Innovative systems to provide building-based potable water and new HVAC technologies to meet changing climates are met by mountains of management overhead. How will tomorrow’s augmented technologies seamlessly integrate into a building?
Perhaps reframing buildings as a problem of organized complexity is the start.
*fin*
Postlude
What are “organized complexity” ways to rethink buildings?
A quote from Gregory Bateson, in Steps to an Ecology of Mind provides us with clues: “Should the original planners put into the very fabric of their plan collateral incentives which will seduce those who come later into carrying out the plan for reasons quite different from those which inspired the plan?”
As we think about embedded economic research queries across space and time: coordination problems, markers of incentive, information sharing despite noise, how might we buy in to the promise of maintenance and adaptability for our future selves… particularly for buildings?
[1] Life and Death of Great American Cities by Jane Jacobs
[2] Read the excellent NAS biographical memoir by Mina Rees, herself an accomplished leader and patron to the sciences– including Deputy Chief of the Applied Mathematics Panel (AMP) with Warren Weaver. More on Mina Rees here
[3] I remember reading that Shannon saw a bulletin job posting about the differential analyzer, and that’s what brought him first to MIT. Anyone know the reference?
[4] Read more in Hunter Heyck’s The Organizational Revolution and Human Sciences
[5] Changed from “A” Mathematical Theory of Communication to “The”
[6] Does that intrigue you? Listen to this 99% Invisible podcast or watch the movie The Sound of Silence for more on the unknown sensory impacts of sound
[7] It’s well known that Jane Jacobs, woman of the details, was at odds with Lewis Mumford, man of the vision. Read Richard Sennett’s Building and Dwelling for a good description of the tension between these two figures.
[8] To quote Herbert Simon in “The Architecture of Complexity“: I shall not undertake a formal definition of complex systems systems thinking. For a comprehensive overview, see Principles of Systems Science by George Mobus
[9] David Mindell would disagree. Check out his excellent book Between Human and Machine: Feedback, Control, and Computing Before Cybernetics for more on the people and innovations that gave rise to the intellectual theory of cybernetics.
[10] Read The Cybernetics Moment by Ronald Kline for a great introduction to the characters of the cybernetics age.
[11] Listen to Ramez Naam on Sean Carroll’s Mindscape podcast for more on carbon-free steel and cement.
And all the links in the text itself!